4.6 billion years ago, in the vicinity of a young sun, a conglomeration of dust and gas coalesced to become the planet Earth. It grew larger due to countless collisions between dust particles, asteroids, and other growing planets, including one final giant impact that threw enough rock, gas, and dust into space to form the moon. The moon was formed when enough rock, gas, and dust were thrown into space to form the moon.
Modern rocks, moon samples, and meteorites help scientists piece together when and how the Earth and moon formed and what they might have looked like in their early stages, even though the rocks that record the earliest parts of Earth’s history have been destroyed or deformed by more than four billion years of geology.
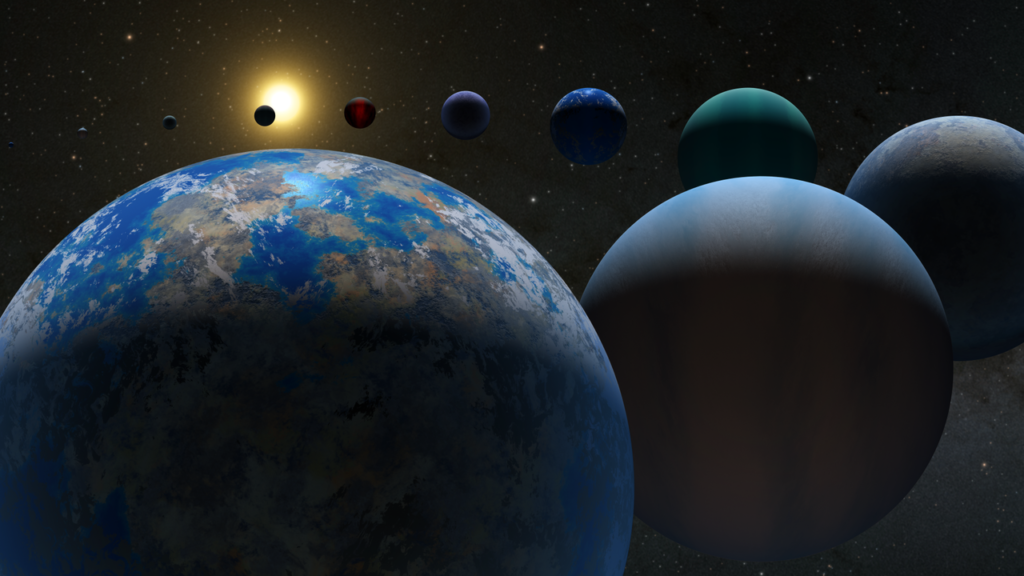
How did the Earth and moon form?
As was the case with all of the other planets in the solar system, the Earth began its existence as a disc of dust and gas that revolved around the infant Sun. It was the forces of drag that brought the dust particles together to form clumps of rock, which eventually grew into “planetesimals” that were tens to hundreds of miles across, and then into “protoplanets” that were the size of Mars by colliding with each other.
The Earth’s final dimensions were achieved as a result of one final catastrophic collision with another Mars-sized object. This final collision, which is also referred to as the “moon-forming impact,” was so massive that, in addition to contributing a significant amount of material to the Earth, there was sufficient energy to vaporise some of the rock and metal from both the proto-Earth and the object that was impacting it. This vapour eventually condensed into a disc around the earth, which eventually became the moon after it cooled down and clumped together.
This information was gleaned from exhaustive research conducted on meteorites and rock samples in the 20th and 21st centuries, some of which took place at the University of Chicago.
To piece together the history of the solar system and find answers to questions like how long it takes for planets to form, what materials planets are made of, and what characteristics make a planet suitable for life, it is essential to have a solid understanding of how the Earth and moon came into existence. Planetary scientists can use this information as a guide in their search for other worlds in our solar system and beyond that might be habitable (or even inhabited!).
When and how did the early Earth come into existence?
New research suggests that Earth’s history began in a disk-shaped cloud of dust and gas rotating around the early sun, composed of remnants from the sun’s own formation, some 4.6 billion years ago.
Gas and dust particles of varying sizes orbited the sun at slightly different speeds within this disc. As a result, these particles were able to collide with one another and bind together. After a period of time, they expanded from the size of minuscule dust grains to that of boulders, and then to that of larger “planetesimals” whose diameters ranged from miles to hundreds of miles.
As they grew larger than the boulders, some planetesimals were able to pull smaller ones out of orbit and absorb them through collisions, causing them to swell to diameters of thousands of miles, or roughly the size of the moon and Mars.
We know ; How.
Meteorites are the critical factor. Meteorites are responsible for bringing a wide variety of materials originating from all over the solar system to Earth, where scientists can study them. These materials consist of chondrules, which are minute fragments of dust and rock that have been preserved from the time before the planets formed, as well as fragments of asteroids and planetesimals that were left behind during the process of planet formation.
When these objects form, radioactive elements like uranium and hafnium are encased within the minerals that make up their composition. This enables planetary scientists to determine the ages of the objects based on the amount of these radioactive elements.
Planetary scientists and astronomers have determined, through the use of these measurements and simulations of the physics of dust and planetesimal collisions, that the process of dust transforming into protoplanets takes tens of millions of years.
On the other hand, the final stage of planet formation in our solar system might have taken a great deal more time – somewhere in the neighbourhood of a hundred million years. Not only was this the last significant addition of material to Earth, but it was also the event that formed the moon. This is one of the aspects of the story that has been the subject of the most debate.
The formation of the moon.
The story that is best supported by all of the available data is that the moon formed during a giant impact between the proto-Earth and another protoplanet roughly the size of Mars, sometimes known as “Theia.” Although scientists have proposed a number of different formation theories for the moon, the story that is best supported by the data is that the moon formed during the collision.
According to this hypothesis, the moon originated from the debris that was thrown into space by the impact, which consisted of molten rock and hot gas at the time. This debris could have potentially formed a disc of material known as a “lunar synestia.”

The following are some alternative hypotheses that have been proposed by scientists:
- “Fission theory” suggests that the moon parted ways with the Earth.
- The “Capture theory” proposes that the moon was formed elsewhere in the solar system and then was “captured” by the gravity of Earth.
- “Co-formation” refers to the simultaneous formation of both the Earth and the moon from the protoplanetary disk.
But how do we know this?
The chemistry of the minerals found in samples of rock brought to Earth by lunar meteorites and the Apollo moon landings can be used to gain a better understanding of the history of the Moon and its connection to the Earth.
Planetary scientists like Prof. Nicolas Dauphas and Prof. Andy Davis in the Geophysical Sciences Department at the University of Chicago make precise measurements of lunar samples to determine exactly what they’re made of, and identify the chemical fingerprints of different geological processes like the melting and mixing of rocks and the evaporation of gasses.
The first big clue about where the moon came from comes from oxygen. Oxygen, like many other elements, is able to exist in multiple forms, known as isotopes. Different types of meteorites coming from the asteroids leftover in the Solar system after planet formation have different proportions of each of these oxygen isotopes. So, by measuring the oxygen isotopes of a given planet, planetary scientists can calculate the different types of asteroid that collided to form the planet. Lunar samples have a very similar oxygen isotope makeup to the Earth.
Oxygen isotopes may indicate that the impactor planet formed relatively close to Earth in the solar system, a theory put forth by some scientists. The object that struck Earth was likely composed of the same mixture of meteorites as Earth itself.
Scientists have also hypothesised that the impact allowed all the oxygen to diffuse freely in the hot vapour surrounding the Earth and moon, thereby erasing the differences between Earth and Theia.
On the other hand, the chemistry of the Earth and the moon are quite different from one another in many respects. Many elements that we typically do not think of as gases, such as potassium, zinc, and sodium, are capable of existing as vapour at the high temperatures that are reached during planetary impacts. These temperatures can reach thousands of degrees Fahrenheit. The amounts of these “volatile” elements found in lunar rocks are significantly lower than those found in rocks found on Earth.
The hot impact debris may have had a considerable amount of time to evaporate these elements before it clumped together to form the moon. This is one of the possibilities. The moon’s low gravity and absence of an atmosphere allowed volatile elements that would not have been able to escape from a larger object to evaporate into space when it first formed. This is another theory that explains the moon’s formation, which occurred at the same time as the Earth and began with a deep magma ocean.
Without referring to a significant influence, these two pieces of evidence are difficult to explain . The high temperatures required to explain the absence of potassium, zinc, and sodium on the moon are provided by an impact origin for the moon. This origin also provides the opportunity for a significant amount of material mixing between the proto-Earth and the material that would eventually become the moon. When exactly did this effect take place, though?
When exactly did the Moon begin to form?
The moon is thought to have been created by a massive collision that occurred between 60 and 175 million years after the birth of the solar system. They are able to arrive at this estimate by using rocks that are found on Earth.
The heat generated by repeated impacts and the radioactive decay of elements within large planetesimals’ minerals is sufficient to cause melting as the planetesimals continue to grow in size. This enables the separation of materials of varying densities, with heavier rocks “floating” on top while denser metals like iron and nickel sink to the centre to form a core and lighter rocks form the outer layer.
The Earth had already begun to separate into these rock and metal layers by the time the impact that formed the moon occurred. However, the proto-Earth was re-melted as a result of the intense force and heat of the impact, which re-mixed the previously separated rock and metal. After this mixing, the Earth was still hot enough for separation to occur again, which resulted in the formation of new rock and metal layers; this is the most important factor to consider when attempting to determine when the moon formed.

Blends of rock and metal allow for the interchange of features from each genre. Elements such as hafnium prefer to be combined with rock rather than metal when possible. Hafnium eventually transforms into tungsten after a period of about 10 million years of radioactive decay. The first time that the Earth cooled down and separated into rock and metal layers was early in the history of the solar system.
As a result, there was a significant amount of hafnium present in the Earth’s rocky layer because it had not yet had the opportunity to decay into tungsten. The majority of this early hafnium had already decayed into tungsten by the time the impact that formed the moon took place. When the impact rearranged the Earth’s crust, the newly formed tungsten gravitated toward the Earth’s metal core because elements like tungsten prefer to be combined with metal. This resulted in the formation of a metal core that contained significantly more tungsten and an outer layer of rocky material that contained a lower concentration of hafnium than it had previously.
Because hafnium has a relatively short half-life in comparison to the age of the Earth, none of it is left on the planet at the present time. However, not everything has been lost, and this fact makes it a very useful tool for determining the order in which events occurred during the first hundred million years of the history of the solar system.
The most recent time that rocks and metals were separated into distinct layers determines when the highest concentration of tungsten was found in the Earth’s rocky strata. The separation of the metal from the rock at an earlier stage cannot account for the extremely low concentration of tungsten found in the Earth’s rocks; therefore, something must have recombined the different layers of the planet. An enormous impact approximately 60-175 million years after the birth of the solar system is the best explanation for the source of the heat and energy that was required to accomplish this.
Just how did the first Earth look like?
Following the collision that gave rise to the moon, Earth was a planet that looked very different from the one we inhabit now. The area of the early Earth that is now covered by oceans was once covered by a magma ocean, which was a layer of molten rock hundreds of miles deep that was melted by the energy released during the collision. The oceans on the modern Earth cover most of the surface of the planet. If there was any water at all, it would only be in the form of water vapour in the air.
On top of that, the sun in those days was a lot more active than it is now, blasting the entire solar system with ultraviolet radiation powerful enough to strip away entire atmospheres.
The Earth’s atmosphere was refilled over time by volcanic eruptions and the water and other gases brought to the surface by comets and meteorites. This occurred after the magma ocean cooled enough to form a solid surface.
This event also marked the beginning of the process by which our planet developed plate tectonics. Plate tectonics is the study of the massive “plates” of crust that slowly move around the surface of the Earth over the course of hundreds of millions of years. This process not only creates new rocks at volcanoes in areas where the plates are moving apart, but it can also recycle rocks from the surface of the Earth and the atmosphere back into the interior of the planet in areas where the plates are moving together.
This process, which is referred to as “subduction,” transports rocks, water, and carbon dioxide that have become trapped in minerals back into the interior of the Earth, where they are capable of driving future volcanic eruptions and continuing the cycle of plate tectonics.
There is a school of thought among planetary scientists that holds that the presence of plate tectonics is necessary for the development of life on a planet. This is due to the fact that the process of plate tectonics, which involves the repeated production and destruction of crust, both releases carbon dioxide to the atmosphere and removes it, which helps keep temperatures on Earth similar (and comfortable for humans, fish, and microbes) over the course of billions of years
However, whether or not a planet has plate tectonics is far more complex than simply determining whether or not it has a solid surface. It may also rely on the types and amounts of various asteroids, planetesimals, and protoplanets that the Earth is made of due to the fact that different substances and minerals can change the manner planet interiors behave over billions of years.
When it was first rising, What did the moon look like?
Although most of us imagine the moon to be a barren, grey landscape dotted with craters and not much else, the moon actually had a surprising amount of geological activity throughout the majority of its history. In the beginning, much like the Earth, the moon had a thick layer of molten rock covering its entire surface.
However, in contrast to the surface of the Earth, the moon’s surface did not cool sufficiently to form tectonic plates. Instead, it possesses a thick crust that is almost entirely composed of feldspar, which is a mineral that has a light colour. The bright areas that are visible on the moon today are referred to as the lunar highlands. The majority of the material that makes up these areas is feldspar. Feldspar began to crystallise as the magma ocean began to cool, and because it was so light, it was able to float to the surface of the moon, on top of other minerals and the magma that was still present.
(Planetary scientists can use the fact that this feldspar crust formed on the moon but not on Earth to attempt to figure out differences in the early chemistry and cooling situations between the two objects in order to find out more about the creation of the moon.) The fact that this feldspar crust formed on the moon but not on Earth can be used by scientists to learn more about the formation of the moon.
However, the formation of a crust composed of feldspar did not mark the end of geological activity on the moon. The heat that was left behind by the impact, in addition to the heat contributed by radioactive elements, was able to melt rock deep within the noon, which provided fuel for volcanoes on the surface. This melting produced basalt, a type of rock with a dark colour that is frequently found at volcanoes on Earth today in places such as Hawaii and Iceland.
The basalt overflowed over hundreds of kilometres across the surface of the moon, forming “mare” (which literally translates to “seas” in Latin) that were up to one mile thick. These mare cover approximately 16% of the surface of the moon and can be seen with the human eye as the dark patches that can be found on the moon.
Based on the number of craters that can be found on each surface type, planetary scientists are able to determine that the feldspar highlands are older than the basalt mare. The mare have fewer craters on their top surfaces than the highlands do because they have been hit by asteroids and meteorites for a shorter period of time than the highlands have. Volcanoes were still erupting on the moon two billion years after the earliest accepted evidence for life on Earth, which means that the youngest mare are only thought to be 1.1 billion years old.
The moon’s orbit was another characteristic of the early moon. The distance between the Earth and the moon continues to increase at a rate of about 1.5 inches per year. Planetary scientists have measured the distance between the Earth and the Moon backwards in time and discovered that the moon used to be seventeen times closer to the Earth (14,000 miles compared to 250,000 miles) when it formed.
Because the size, speed, and angles of approach of impactors in moon formation simulations change the orbit of the final Earth-moon system, this fluctuating distance between the Earth and the moon is an important clue about the specifics of the impact that formed the moon. Planetary scientists need to devise a simulation of an impact that not only replicates the moon’s chemical composition but also accounts for the moon’s distance from Earth and its initial rate of rotation when it was formed.
Still some questions to be answered.
There is a consensus among scientists that the moon was created as a result of a collision with another object; however, the specifics of this collision are not yet fully understood. There is still a lack of consensus among scientists regarding the size of the object that caused the impact, the speed at which it was travelling, the composition of the object, and even whether or not we should call it “Theia.” There are even those among the scientific community who contend that the Moon may have been formed by more than one collision.
Experiments conducted in labs help scientists gain a better understanding of what happens to various types of rocks and elements when subjected to the extreme conditions that are present during large impact events. At the University of Chicago, the research group led by Professor Nicolas Dauphas evaporates metals in a vacuum to recreate the conditions that existed in the cloud of impact debris. This is done in an effort to provide an explanation for why lunar rocks contain a significantly lower concentration of elements such as sodium, zinc, and potassium in comparison to rocks found on Earth.
However, given that some experiments may be too big for the laboratory, computer simulations are also utilised in the investigation of the impact that led to the formation of the moon. With the help of these simulations, scientists are able to virtually collide the proto-Earth with various kinds of planetesimals at a variety of speeds and angles in order to determine which combinations of characteristics are capable of producing a moon with the size and orbit that we observe currently.
New samples brought back from the moon in the future might provide planetary scientists with access to a greater variety of lunar rock types for research purposes. This is significant because it’s possible that new samples will record different aspects of the history of the moon that aren’t found in the lunar rocks that were brought back from previous Apollo missions. Planetary scientists will also be able to measure new chemical signatures in lunar rocks as measurement techniques continue to advance, allowing them to improve upon previously obtained data and make new discoveries.
The more measurements that scientists have, the more ways there are for them to test different theories about how our moon formed, its relationship to the Earth, and possibly even how moons might form around other planets that are located outside of our solar system!